- HEAT ENERGY, CONCEPT OF HEAT AND TEMPERATURE
- THERMOMETER (TYPES AND CALCULATION)
- EXPANSIVITY
- EXPANSIVITY
- HEAT TRANSFER
- ELECTRIC CHARGES PRODUCTION
- GOLD LEAF ELECTROSCOPE
- FIELD CONCEPT AND TYPES OF FIELD
- ELECTRIC FIELD
- PRODUCTION OF CONTINUOUS ELECTRIC CURRENT, CHEMICAL ENERGY, HEAT ENERGY, MECHANICAL ENERGY, SOLAR ENERGY
HEAT ENERGY, CONCEPT OF HEAT AND TEMPERATURE
Physics, SS 1, week 1
Topic: HEAT ENERGY, CONCEPT OF HEAT AND TEMPERATURE. EFFECTS OF HEAT: CHANGE OF STATE/ PHASE RISE/FALL IN TEMPERATURE EXPANSION/CONTRACTION, CHANGE OF RESISTANCE, SOURCE, USES OF HEAT.
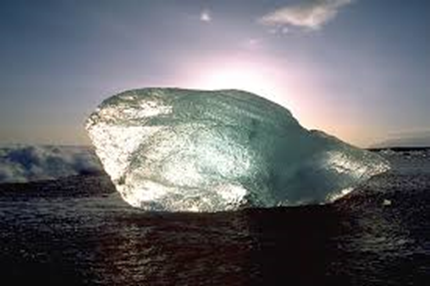
knowing the difference between heat and temperature is important. It can lead to a clearer understanding of energy. Above is a picture of an ice cube melting in a small dish. The ice, water, dish, and are experience heat exchanges and temperature changes. In this section we will define both heat and temperature and hopefully reach an understanding of how they are related, but not identical ideas. What follows are introductions.
Often the concepts of heat and temperature are thought to be the same, but they are not.
Perhaps the reason the two are usually and incorrectly thought to be the same is because as human beings on Earth our everyday experience leads us to notice that when you add heat to something, say like putting a pot of water on the stove, then the temperature of that something goes up. More heat, more temperature – they must be the same, right? Turns out, though, this is not true.
Initial Definitions
Temperature is a number. That number is related to energy, but it is not energy itself.
Temperature is a number that is related to the average kinetic energy of the molecules of a substance.
Read that last sentence carefully. It does not say that temperature is kinetic energy, nor does it state exactly what is the relation between temperature and kinetic energy?
Here is the relation: If temperature is measured in Kelvin degrees, then its value is directly proportional to the average kinetic energy of the molecules of a substance. Note that temperature is not energy, it is a number proportional to a type of energy.
Heat, on the other hand, is actual energy measured in Joules or other energy units. Heat is a measurement of some of the energy in a substance. When you add heat to a substance, you are adding energy to the substance. This added heat (energy) is usually expressed as an increase in the kinetic energies of the molecules of the substance. If the heat (energy) is used to change the state of the substance, say by melting it, then the added energy is used to break the bonds between the molecules rather than changing their kinetic energy.
So, temperature is not energy. It is, though, a number that relates to a type of energy possessed by the molecules of a substance. Temperature directly relates to the kinetic energy of the molecules.
Temperature can be measured in a variety of units.
If you measure it in degrees Kelvin, then the temperature value is directly proportional to the average kinetic energy of the molecules in the substance.
Notice we did not say that temperature is the kinetic energy. We said it is a number, if in degrees Kelvin, that is proportional to the average kinetic energy of the molecules of a substance. That means if you double the Kelvin temperature of a substance, you double the average kinetic energy of its molecules.
When the average kinetic energy of the molecules goes up (a rise in temperature), the average speed of the molecules increases. A change in average kinetic energy is not directly proportional to a change in average speed.
More about Heat
Heat is energy. When you add heat to a substance, you are adding energy.
When heat (energy) goes into a substance one of two things can happen:
1. The substance can experience a rise in temperature. The heat (the added energy) can be realized as an increase in the average kinetic energy of the molecules. The molecules now, on average, have more kinetic energy. This increase in average kinetic energy is registered as a number called temperature that changes proportionally with it. Note that this increase in the average kinetic energy of the molecules means that they will now, on average, be traveling faster than before the heat arrived.
2. The substance can change state. For example, if the substance is ice, it can melt into water. Perhaps surprisingly, this change does not cause a rise in temperature. At the exact moment before melting, the average kinetic energy of the ice molecules is the same as the average kinetic energy of the water molecules at the exact moment after melting. That is, the melting ice and the just melted water are at the same temperature. Although heat (energy) is absorbed by this change of state, the absorbed energy is not used to change the average kinetic energy of the molecules, and thus proportionally change the temperature. The energy is used to change the bonding between the molecules. Changing the manner in which the molecules bond to one another can require absorption of energy (heat) as in the case of melting, or require a release of energy (heat) as in the case of freezing.
So, when heat comes into a substance, energy comes into a substance. That energy can be used to increase the kinetic energy of the molecules, which means an increase in their temperature which means an increase in their speed. Or at certain temperatures the added heat could be used to break the bonds between the molecules causing a change in state that is not accompanied by a change in temperature.
CHANGES OF STATE/CHANGES OF PHASE
The term ‘change of phase’ means the same thing as the term ‘change of state’.
There are four states, or phases, of matter. They are:
- Solid
- Liquid
- Gas
- Plasma
We will not be discussing the plasma state here.
When a substance changes from one state, or phase, of matter to another we say that it has undergone a change of state, or we say that it has undergone a change of phase.
These changes of phase always occur with a change of heat. Heat, which is energy, either comes into the material during a change of phase or heat comes out of the material during this change. However, although the heat content of the material changes, the temperature does not.
Here are the five changes of phase, listed below:
Description of Phase Change | Term for Phase Change | Heat Movement During Phase Change | Temperature Change During Phase Change |
Solid to liquid | Melting/Fusion | Heat goes into the solid as it melts. | None |
Liquid to solid | Freezing | Heat leaves the liquid as it freezes. | None |
Liquid to gas | Vaporization, which includes boiling and evaporation | Heat goes into the liquid as it vaporizes. | None |
Gas to liquid | Condensation | Heat leaves the gas as it condenses. | None |
Solid to gas | Sublimation | Heat goes into the solid as it sublimates. | None |
So, how could there be a change in heat during a state change without a change in temperature? During a change in state the heat energy is used to change the bonding between the molecules. In the case of melting, added energy is used to break the bonds between the molecules. In the case of freezing, energy is subtracted as the molecules bond to one another. These energy exchanges are not changes in kinetic energy. They are changes in bonding energy between the molecules.
If heat is coming into a substance during a phase change, then this energy is used to break the bonds between the molecules of the substance. Whenever ice melts into water, immediately after the molecular bonds in the ice are broken the molecules are moving at the same average speed as before, so their average kinetic energy remains the same, and, thus, their Kelvin temperature remains the same.
The molecule of ice and the molecule of water move with the same rate of vibration. This is meant to show that they have the same average speed and thus the same average kinetic energy (since they have the same mass) and thus the same Kelvin temperature. The motions are, though, greatly exaggerated. Actually, the motions of the molecules should be considered tiny vibrations.
In the ice the molecules are strongly bonded to one another, thus forming a rigid solid. When heat is added to the ice it melts, and these bonds are broken, The molecules afterward bond to one another with less strength, and water is formed.
Now, before the melting, the molecules were actually moving when in the solid state. They were vibrating back and forth. They had an average kinetic energy. So they had a Kelvin temperature proportional to this average kinetic energy.
After the melting the water molecules are moving, also. And they have the same average kinetic energy as they had before the melting. So, the water is at the same temperature the moment after the melting that the ice was at the moment before the melting.
Heat came into the situation, but it was not used to change the kinetic energy of the molecules. It was used to change the bonding between the molecules. Breaking the bonds between the molecules of the ice requires energy, and this energy is the added heat.
In a similar way heat enters a liquid to change the molecular bonding when the liquid boils or evaporates into a gas, and heat enters a solid to change the molecular bonding when it sublimates into a gas.
In an inverse way heat leaves a gas to change the molecular bonding when the gas condenses into a liquid, and heat leaves a liquid to change the molecular bonding when it freezes into a solid.
In none of these changes of state is the heat (energy) that is input or output used to change the speed of the molecules. The average speed of the molecules is the same before and after a phase change, and so is the average kinetic energy. And so, again, note that the temperature does not change during a change in phase, since it is proportional, in Kelvin degrees, to the average kinetic energy, which does not change.
Sources and Uses
We consume energy in dozens of forms. Yet virtually all of the energy we use originates in the power of the atom. Nuclear reactions energize stars, including our sun. The energy we capture for use on Earth comes largely from the sun or from nuclear forces local to our own planet.
Sunlight is by far the predominant source, and it contains a surprisingly large amount of energy. On average, even after passing through hundreds of kilometers of air on a clear day, solar radiation reaches Earth with more than enough energy in a single square meter to illuminate five 60-watt light bulbs if all the sunlight could be captured and converted to electricity.
The sun’s energy warms the planet’s surface, powering titanic transfers of heat and pressure in weather patterns and ocean currents. The resulting air currents drive wind turbines. Solar energy also evaporates water that falls as rain and builds up behind dams, where its motion is used to generate electricity via hydropower.
Most Americans, however, use solar energy in its secondhand form: fossil fuels. When sunlight strikes a plant, some of the energy is trapped through photosynthesis and is stored in chemical bonds as the plant grows. We can recover that energy months or years later by burning wood, which breaks the bonds and releases energy as heat and light. More often, though, we use the stored energy in the much more concentrated forms that result when organic matter, after millions of years of geological and chemical activity underground, turns into fossil fuels, such as coal, oil, or natural gas. Either way, we’re reclaiming the power of sunlight.
The only other original source of energy on Earth’s surface is found in more local nuclear reactions, where atoms of radioactive elements such as uranium split apart into smaller atoms and liberate energy in the process. Harnessed as heat, the released energy boils water, producing steam that turns turbines, thereby being converted to mechanical energy that generates electricity. Nuclear energy currently provides 20% of total electricity generation in the United States.
Finally, the heat of Earth’s molten interior, itself largely the result of the nuclear decay of radioactive elements, provides geothermal energy. At present, it is chiefly used in only a few places, such as California and Iceland, where proximity to high temperature geothermal fields makes it practical.
Question:
1. Temperature is not energy; it is …………………………….. proportional to a type of energy.
A. Gas B. A Form C. A Number D. geologically
2. Freezing is the conversion from
A. Liquid to solid B. Solid to gas C. Gas to liquid D. Liquid to gas
3. Heat enters a liquid to change the …………………………… when the liquid boils or evaporates into a gas.
A. Atomic bonding B. Molecular bonding C. Nuclear Bonding D. Chemical bonding
4. The predominant source of energy in this 21st century is from the.
A. Nuclear reactor B. Sunlight C. Sea D. Ground
5. Heat leaves a gas to change the molecular bonding when the gas condenses into a liquid.
A. Liquid B. Solid C. Steam D.
Answers:
1. C 2. A 3. B 4. B 5. A
THERMOMETER (TYPES AND CALCULATION)
Physics, SS 1 week 2
Topic: Evolution of the Thermometer
A thermometer is a device that gauges temperature by measuring a temperature-dependent property, such as the expansion of a liquid in a sealed tube. The Greco-Roman physician Galen (c. 129-c. 199) was among the first thinkers to envision a scale for measuring temperature, but development of a practical temperature-measuring device—the thermoscope—did not occur until the sixteenth century.
The great physicist Galileo Galilei (1564-1642) may have invented the thermoscope; certainly he constructed one. Galileo’s thermoscope consisted of a long glass tube planted in a container of liquid. Prior to inserting the tube into the liquid—which was usually colored water, though Galileo’s thermoscope used wine—as much air as possible was removed from the tube. This created a vacuum (an area devoid of matter, including air), and as a result of pressure differences between the liquid and the interior of the thermoscope tube, some of the liquid went into the tube.
But the liquid was not the thermometric medium—that is, the substance whose temperature-dependent property changes were measured by the thermoscope. (Mercury, for instance, is the thermometric medium in many thermometers today; however, due to the toxic quality of mercury, an effort is underway to remove mercury thermometers from U.S. schools.) Instead, the air was the medium whose changes the thermoscope measured: when it was warm, the air expanded, pushing down on the liquid; and when the air cooled, it contracted, allowing the liquid to rise.
Early Thermometers: The Search for a Temperature Scale
The first true thermometer, built by Ferdinand II, Grand Duke of Tuscany (1610-1670) in 1641, used alcohol sealed in glass. The latter was marked with a temperature scale containing 50 units, but did not designate a value for zero. In 1664, English physicist Robert Hooke (1635-1703) created a thermometer with a scale divided into units equal to about 1/500 of the volume of the thermometric medium. For the zero point, Hooke chose the temperature at which water freezes, thus establishing a standard still used today in the Fahrenheit and Celsius scales.
Olaus Roemer (1644-1710), a Danish astronomer, introduced another important standard. Roemer’s thermometer, built in 1702, was based not on one but two fixed points, which he designated as the temperature of snow or crushed ice on the one hand, and the boiling point of water on the other. As with Hooke’s use of the freezing point, Roemer’s idea of designating the freezing and boiling points of water as the two parameters for temperature measurements has remained in use ever since.
How a Thermometer Works
Today, there are a variety of types of thermometers. The type that most of us are familiar with from science class is the type that consists of a liquid encased in a narrow glass column. Older thermometers of this type used liquid mercury. In response to our understanding of the health concerns associated with mercury exposure, these types of thermometers usually use some type of liquid alcohol. These liquid thermometers are based on the principle of thermal expansion. When a substance gets hotter, it expands to a greater volume. Nearly all substances exhibit this behavior of thermal expansion. It is the basis of the design and operation of thermometers.
As the temperature of the liquid in a thermometer increases, its volume increases. The liquid is enclosed in a tall, narrow glass (or plastic) column with a constant cross-sectional area. The increase in volume is thus due to a change in height of the liquid within the column. The increase in volume, and thus in the height of the liquid column, is proportional to the increase in temperature. Suppose that a 10-degree increase in temperature causes a 1-cm increase in the column’s height. Then a 20-degree increase in temperature will cause a 2-cm increase in the column’s height. And a 30-degree increase in temperature will causes 3-cm increase in the column’s height. The relationship between the temperature and the column’s height is linear over the small temperature range for which the thermometer is used. This linear relationship makes the calibration of a thermometer a relatively easy task.
The calibration of any measuring tool involves the placement of divisions or marks upon the tool to measure a quantity accurately in comparison to known standards. Any measuring tool – even a meter stick – must be calibrated. The tool needs divisions or markings; for instance, a meter stick typically has markings every 1-cm apart or every 1-mm apart. These markings must be accurately placed and the accuracy of their placement can only be judged when comparing it to another object known to have an accurate length.
A thermometer is calibrated by using two objects of known temperatures. The typical process involves using the freezing point and the boiling point of water. Water is known to freeze at 0°C and to boil at 100°C at an atmospheric pressure of 1 atm. By placing a thermometer in mixture of ice water and allowing the thermometer liquid to reach a stable height, the 0-degree mark can be placed upon the thermometer. Similarly, by placing the thermometer in boiling water (at 1 atm of pressure) and allowing the liquid level to reach a stable height, the 100-degree mark can be placed upon the thermometer. With these two markings placed upon the thermometer, 100 equally spaced divisions can be placed between them to represent the 1-degree marks. Since there is a linear relationship between the temperature and the height of the liquid, the divisions between 0 degree and 100 degree can be equally spaced. With a calibrated thermometer, accurate measurements can be made of the temperature of any object within the temperature range for which it has been calibrated.
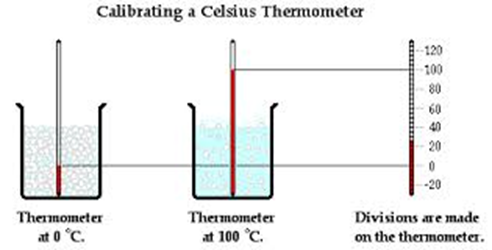
Temperature Scales
The thermometer calibration process described above results in what is known as a centigrade thermometer. A centigrade thermometer has 100 divisions or intervals between the normal freezing point and the normal boiling point of water. Today, the centigrade scale is known as the Celsius scale, named after the Swedish astronomer Anders Celsius who is credited with its development. The Celsius scale is the most widely accepted temperature scale used throughout the world. It is the standard unit of temperature measurement in nearly all countries, the most notable exception being the United States. Using this scale, a temperature of 28 degrees Celsius is abbreviated as 28°C.
Traditionally slow to adopt the metric system and other accepted units of measurements, the United States more commonly uses the Fahrenheit temperature scale. A thermometer can be calibrated using the Fahrenheit scale in a similar manner as was described above. The difference is that the normal freezing point of water is designated as 32 degrees and the normal boiling point of water is designated as 212 degrees in the Fahrenheit scale. As such, there are 180 divisions or intervals between these two temperatures when using the Fahrenheit scale. The Fahrenheit scale is named in honor of German physicist Daniel Fahrenheit. A temperature of 76 degree Fahrenheit is abbreviated as 76°F. In most countries throughout the world, the Fahrenheit scale has been replaced by the use of the Celsius scale.
Temperatures expressed by the Fahrenheit scale can be converted to the Celsius scale equivalent using the equation below:
°C = (°F – 32°)/1.8
Similarly, temperatures expressed by the Celsius scale can be converted to the Fahrenheit scale equivalent using the equation below:
°F= 1.8•°C + 32°
The Kelvin Temperature Scale
While the Celsius and Fahrenheit scales are the most widely used temperature scales, there are several other scales that have been used throughout history. For example, there is the Rankine scale, the Newton scale and the Romer scale, all of which are rarely used. Finally, there is the Kelvin temperature scale, which is the standard metric system of temperature measurement and perhaps the most widely used temperature scale used among scientists. The Kelvin temperature scale is similar to the Celsius temperature scale in the sense that there are 100 equal degree increments between the normal freezing point and the normal boiling point of water. However, the zero-degree mark on the Kelvin temperature scale is 273.15 units cooler than it is on the Celsius scale. So a temperature of 0 Kelvin is equivalent to a temperature of -273.15 °C. Observe that the degree symbol is not used with this system. So a temperature of 300 units above 0 Kelvin is referred to as 300 Kelvin and not 300 degree Kelvin; such a temperature is abbreviated as 300 K. Conversions between Celsius temperatures and Kelvin temperatures (and vice versa) can be performed using one of the two equations below.
°C = K – 273.15°
K = °C + 273.15
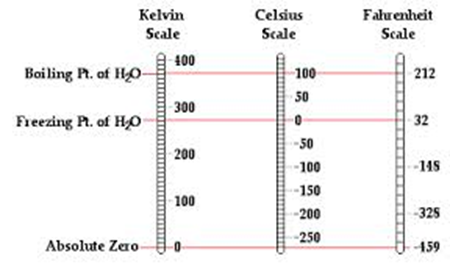
The zero point on the Kelvin scale is known as absolute zero. It is the lowest temperature that can be achieved. The concept of an absolute temperature minimum was promoted by Scottish physicist William Thomson (a.k.a. Lord Kelvin) in 1848. Thomson theorized based on thermodynamic principles that the lowest temperature which could be achieved was -273°C. Prior to Thomson, experimentalists such as Robert Boyle (late 17th century) were well aware of the observation that the volume (and even the pressure) of a sample of gas was dependent upon its temperature. Measurements of the variations of pressure and volume with changes in the temperature could be made and plotted. Plots of volume vs. temperature (at constant pressure) and pressure vs. temperature (at constant volume) reflected the same conclusion – the volume and the pressure of a gas reduces to zero at a temperature of -273°C. Since these are the lowest values of volume and pressure that are possible, it is reasonable to conclude that -273°C was the lowest temperature that was possible.
Thomson referred to this minimum lowest temperature as absolute zero and argued that a temperature scale be adopted that had absolute zero as the lowest value on the scale. Today, that temperature scale bears his name. Scientists and engineers have been able to cool matter down to temperatures close to -273.15°C, but never below it. In the process of cooling matter to temperatures close to absolute zero, a variety of unusual properties have been observed. These properties include superconductivity, super fluidity and a state of matter known as a Bose-Einstein condensate.
Questions:
1. Principle of thermal expansion; when a substance gets hotter
A. It expands to a greater volume B. It expands to a greater mass C. It expands to a greater pressure D. It contracts to a lesser mass.
2. Which is correct, conversion between Celsius temperatures and Kelvin temperatures?
- °C = K + 273.15° B. °C = K – 237.15° C. °C = K – 273.15° D. °C = K +237.15°
3. Temperatures expressed by the Fahrenheit scale can be converted to the Celsius scale equivalent using the equation below:
- °C = (°F – 32°)/1.6 B . °C = (°F – 32°)/1.8 C. °C = (°F + 32°)/1.7 C. °C = (°F + 32°)/1.8
4. A thermometer is a device that …………….. temperature by measuring a temperature-dependent property.
A. distributes B. reduces C. gauges D. manipulates
5. The first true thermometer, built by Ferdinand II, Grand Duke of Tuscany (1610-1670) in 1641, used ………………… sealed in glass.
A.alcohol B. nitric acid C. ammonium D. water
Answers:
1. A 2. C 3. B 4. B 5. A
EXPANSIVITY
Physics, SS 1 week 3
Topic: Expansivity
When heated, most solids and liquids expand. They almost contract when cooled. Expansion means an increase in the size of an object.
According to the kinetic molecular theory, when an object is heated the molecules acquire more kinetic energies which enables them to overcome their intermolecular forces. Therefore the vibrations of the molecules increase and their displacements about their mean positions increase.
As a result of this, the average distance between the molecules of the substance becomes larger reading to an increase in the size of the substance. This increase in the dimension of the heated object depends on the strength of the intermolecular forces. If these forces are stronger, the expansion will be small and vice versa.
The intermolecular forces are stronger in solids than in liquids and weakest in gases. Hence when heat is applied, gases expand more than liquids and liquids expand more than solids.
Each particular substance has an intermolecular force peculiar to it. Therefore the addition of heat causes different expansions in different substances.
Advantages of expansion
i. Red-hot rivets in ship
ii. Removal of tight glass stopper
iii. Fire-alarms e.g. electric bell
iv. Fitting of wheels in rims
v. Expansion of metals used in bimetallic thermometer
vi. Bimetallic strip used in thermostat
Disadvantages of expansion
i. Expansion of metal or concrete bridges
ii. Cracking of glass cup when hot water is poured into it
iii. Expansion of balance wheel or wrist-watch
iv. Sagging of overhead – wire
v. Expansion of railway lines
vi. Bursting of water pipes.
Expansion in Solid
Solids expand when heated and contract when cooled. We can demonstrate this by the ‘ball and ring experiment’.
Ball and Ring Experiment
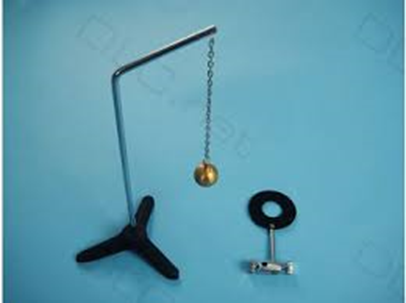
In experiments, the ball and ring experiment is a volume expansion demonstration experiment in which: (a) a cold (room temperature) brass ball is shown to be able to pass through tightly-fitted brass ring, (b) the ball is then heated with a flame, and (c) the experimenter then shows that the hot steel ball is unable to fit through the ring, as it previous was able to before, in the cold state, thus providing experimental proof that bodies do measurably expand when heated.
A circa 1900 version of the ring and ball experiment, is shown adjacent, wherein, a brass ball with a diameter of 29 mm, at height 260 mm, when heated will not pass through the brass ring, but when cold or ambient temperature will.
Steel rod Experiment
This experiment shows how a solid expands when subjected to a particular temperature and contracts when cooled. Consider a rod clamped on a retort stand so that its order end is just on an insulator and allowed to roll on. When it is heated, the rod expands away from its initial point on the rule, which can be seen as the pointer moves and when the flame is removed, the rod contracts and the pointer returns to its original position. This shows expansion when heated and contraction when cooled.
Application of Expansion
1. Railway tracks: Railway tracks have gaps to make allowance for expansion due to a rise in temperature, otherwise the rails would buckle. In past years, many miles of railway tracks have been replaced with long sections of continuously welded track. The old track had fish-plate junctions at fairly short intervals. A “clickety-click” effect was then felt as the train passed over the jointed parts. The newer and safer track has long rail lengths joined by rigid welds. These are flat with the rail surfaces to give a much smoother train ride.
Bimetal Strips: A bimetal strip is heated, it curves with the brass outside of the curve. This shows that brass expands more than iron for the same temperature rise. The bimetal strip straightens when it cools to room temperature. If it cooled below room temperature, the brass would contract more than the iron. So, the strip would curve with the iron on the outside of the curve.
Rivets: Steel plate such as those used in sheep building or in large boilers are usually riveted together using red-hot rivets. Hotels are made in the overlapping plates, A and C, a red-hot rivet is pushed through and its head, H, held tightly against plate A. The other end B of the rivet is hammered tight against the other plate C. On cooling, the rivet contracts and holds the plates even more tightly together. This provides a good seal against the sea for ship plates and against steam in large boilers.
ASSESSMENT
- When water is heated from 0º C to 20º C its volume
(a) goes on increasing
(b) goes on decreasing
(c) remains constant up to 15º C and then increases
(d) first decreases and then increases
(e) remains constant up to 4º C and then increases - 5 g of ice at 0º C is mixed with 10 g of water at 10º C. The temperature of the mixture is
(a) 0º C
(b) 2º C
(c) 2.5º C
(d) 5º C
(e) 7.5º C - Equal masses of three liquids of specific heats C1, C2and C3 at temperatures t1, t2and t3 respectivelyare mixed. If there is no change of state, the temperature of the mixture is
(a) (t1+ t2+ t3)/3
(b) (C1t1+ C2t2+ C3t3)/[3(C1+ C2+ C3)]
(c) (C1t1+ C2t2+ C3t3)/ (C1+ C2+ C3)
(d) 3(C1t1+ C2t2+ C3t3)/ (C1+ C2+ C3)
(e) 3(t1+ t2+ t3) - The amount of heat required to raise the temperature of one mole of an ideal mono atomic gas through 2º C at constant pressure is (universal gas constant = R)
(a) 2 R
(b) 3 R
(c) 5 R
(d) 5R/2
(e) 7R/2 - (5) Two identical rectangular strips, one of copper and the other of steel, are riveted as shown to form a bi-metal strip. On heating, the bi-metal strip will
(a) get twisted
(b) remain straight
(c) bend with steel on the convex side
(d) bend with steel on the concave side
(e) contract
ANSWERS
- d
- a
- d
- c
- d
EXPANSIVITY
Physics SS 1 week 4
Topic: EXPANSIVITY
When a solid is heated is to a particular temperature, the molecule it contains absorb some kinetic energy, since kinetic energy is the measure of velocity (i.e. the higher the kinetic energy, the higher the velocity of the molecules). These molecules move faster and collide with one another and with the wall of the system to the event that the strong molecular force of attraction is broken after several collisions and by so doing the system expands. Linear expansivity of solid (metal)is defined as the increase in length per unit rise in temperature. It is denoted by α and measured in K-1 or 0C-1 (SI unit).
Original length at to1C = Lo
Final length when heated at to1 C = Lo
Final length when heated at to2C = Lt
Linear expansivity/coefficient of expansion = α
Change in temperature from t2 – t1 = ∆θ.
α = L2 – L1 / L1∆θ
L2 – L1 = L1α∆θ
L2 = L1 (1 + α∆θ)
Example: If a metal rod of length 50m expands when heated at a temperature change of 10k, find the charge in length (linear expansivity of metal), [α = 1.5 x 10-8 k-1]
Solution:
Using α = L2 – L1 / L1 ∆t given that L1 = 50m, t = 10k
∆L = L2 – L1 = L1 ∆t α = 1.5 x 10-8 k-1
∆L = L2 – L1 = 1.5 x 10-8 x 10 x 50
∆L = L2 – L1 = 750 x 10-8
∆L = L2 – L1 = 7.5 x 10-6m
Change in length is 7.5 x 10-6m
Are and Volume expansivity
When a solid is heated, it expands in all directions – in length, in breath and in height. Hence there is an increase in the area as well as in the volume of the solid. The increase in area when a body is heated is known as area or superficial expansion.
The area or superficial expansivity, ß, of a solid is the increase in area per unit area degree Kelvin increase in temperature or the fractional increase in area per Kelvin rise in temperature.
Similarly, an increase in volume when a body is heated is known as cubic or volume expansion and we define expansivity as follows:
The volume or cubic expansivity, y, is the increase in volume of a substance per unit volume per Kelvin rise in temperature or the fractional increase in volume per Kelvin rise in temperature.
Area expansivity ß
= change in area/original area x temperature rise
ß = A2 – A1 / A1 x θ
where A2 = area at temperature θ2
A1 = area at temperature θ1
θ = θ2 – θ1
A2 = A1(1 + ßθ)
Increase in area = A2 – A1=A1 ßθ
Volume Thermal Expansion: When the temperature of a volume changed ΔT, the change of its volume ΔV is very nearly proportional to its initial volume multiplied by ΔT. The Volume Expansion equation is:
ΔV = βV0ΔT
Where:
β: the Coefficient of volume expansion
V0: Initial volume of the object
ΔV: Volume change of the object
ΔT: Temperature change of the object
We have seen that change in length (or volume) with changing temperature can be used to create a thermometer. The old-fashioned mercury in glass thermometer relies on this phenomenon.
But exactly how much does an object change its length (or volume) as the temperature changes? Experimentally we find that for a solid or liquid, the length change is given by,
∆l = αl0∆T
Where l is the “original” length, ∆T is the change in temperature and α is the coefficient of linear expansion. The coefficient of linear expansion is approximately independent of temperature and is very small, e.g. for steel α = 12 x 10-6 K-1.
Linear expansion is important only when one dimension of an object is much larger than the other two. For volume expansion it can be shown that,
∆V = ßV0∆T
where V0 is the “original” volume and ß = 3αis the volume coefficient of expansion.
Anomalous Expansion of Water
Water is a unique material in many ways. Without it and its unusual properties life as we know it could not exist. One of these critical properties is the so-called anomalous expansion. When heated, most substances expand according to the simple description above, but when heated through the temperature range 0 – 4 0C water contracts (its density increases).
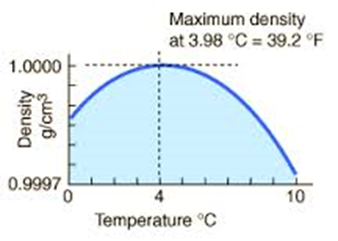
Furthermore, as you are probably aware, when water is cooled and freezes (at 0 0C) the frozen water (ice) expands becoming less dense, causing problems with roads, buildings etc. This property is very unusual, most substances, on freezing contract rather than expand. This property of water explains why ice floats. If this were not the case, the ice created in the winter would sink to the bottom of lakes and oceans and would be insulated from melting in the summer. After a short time our oceans and lakes would consist of a solid mass of ice with only a thin layer at the surface alternately freezing and melting as the seasons came and went – hardly conducive to the development of life.
Expansion of Liquid
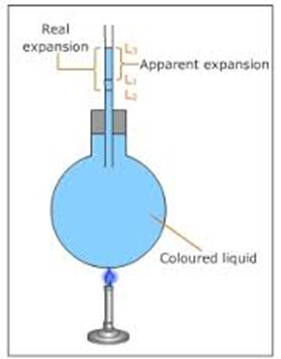
Liquid Expansion
Real expansivity of liquid
Real expansivity of liquid is sometimes called cubic expansivity of liquid and it is defined as the increase in volume per unit degree rise in temperature. Since liquid does not have a particular length or area, then we talk of its volume about its container.
The cubic expansivity is sometimes called the real expansivity yr
When a liquid is heated in a vessel, expansion occurs both in the liquid and in the vessel. Then from the vessel we have apparent expansivity of the liquid.
Apparent cubic expansivity
Apparent cubic expansivity of a liquid is define as the mass of the liquid expelled per unit divided by mass left or remaining when the temperature increases by 1oC. It is measured in K-1.
Thus,
Apparent cubic expansivity = mass of liquid expelled/mass of liquid left x temperature rise.
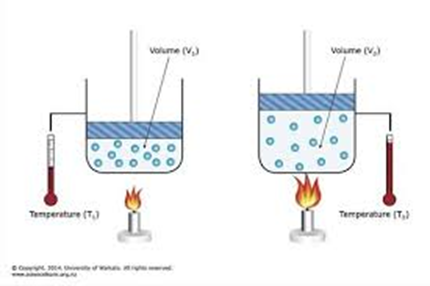
Expansion of various liquids
ASSESSMENT
- On heating, amplitude of vibration of atoms or molecules of an object
(a) increases
(b) decreases
(c) remains constant
(d) none of above - Melting point of water is
(a) 323 °C
(b) 100 °C
(c) 273 °C
(d) 0 °C - Melting point is also known as
(a) fusion point
(b) constant point
(c) boiling point
(d) freezing point - Coefficient of volume expansion of solids is
(a) greater than liquids
(c) equal to gases
(c) less than liquids
(d) equal to liquids - Temperature of land rises more quickly than that of sea because
(a) specific heat of soil is more than water
(b) specific heat of soil is less than water
(c) soil is solid
(d) none of above
ANSWERS
- a
- d
- a
- b
- c
HEAT TRANSFER
WEEK 5
Topic: HEAT TRANSFER
Heat flows from a body at higher temperature to another at a lower temperature to another at a lower temperature. This transfer of heat can happen in three ways: conduction, convection and radiation.
Conduction
It is our everyday experience but when we pour hot water into an aluminum cup, the handle of the cup soon feels hot to the touch. If you deep a silver spoon into a hot tea, the handle of the spoon very quickly feels hotter than it was before. The heat from the hot water has been transferred along the metal handle to the other end of the cup or spoon by the process of conduction.
Conduction of heat is the process by which heat energy is transferred through a metal, the average position of the particles of the material remaining the same.
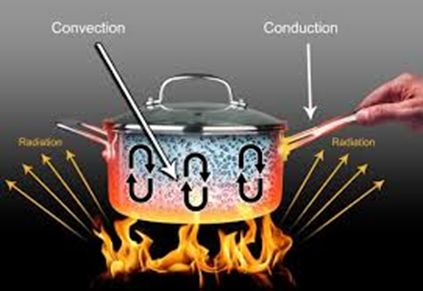
Heat energy is always transferred when different parts of a solid body are at different temperatures. The direction of heat transfer is always from the hotter to the cooler parts of the solid. For example, for the spoon dipped into a hot tea, the heat is transferred for the bowl of the spoon inside the hot tea to the handle of the spoon outside the tea.
Most metals (e.g copper, aluminium, silver, iron) allow heat energy to pass through them very easily. Such materials are known as good conductors of heat. Most non-metals (e.g. water, air, wood, plastic, cloth, cotton, wool, cork) are poor conductors of heat or insulators. Such materials do not allow heat to pass easily through them. The handle of a cooking utensil is made of wood or plastic which are insulators, but the cooking utensil itself is made of a good conductor (e.g. aluminum), so that heat can be conducted quickly through utensil but not through the handle. The ability of a metal to conduct heat is known as its thermal conductivity.
Practical application of good and bad conductors
1. Cooking Utensils
Good conductors are used whether it is necessary to conduct heat quickly. Thus cooking pot and frying pan are usually made of metals such as aluminium which is a good conductor. Thus ensure quick transfer of heat from the fire to the food being cooked, and a quick cooking of the food.
On the other hand, the handles of cooking utensils are made of insulators like wood or plastic materials, so that the utensils when hot can be held comfortably by the handle.
2. Use of rugs on floors
A tile floor is much colder to the feet than a rug covered floor at the same temperature. This is because tile is a better conductor of heat than rug. Heat transferred from one foot to the rug is not conducted away rapidly, so the rug quickly heats up on its surface to the temperature of the foot; but the tile conducts the heat away rapidly from one’s foot, and thus can take more heat from the foot, so its surface temperature drops and the foot feels cold.
3. Home Cooling in the Tropics
It is our common experience that thatched-roofed houses are cooler inside than galvanized iron roofed ones, especially in the absence of asbestos ceilings. The iron conducts the heat from the sun and heats up inside the house. To reduce this heat asbestos ceilings are used. Asbestos is an insulator and prevents the heating up of the house. Thatched-roofed houses need no ceilings to maintain cool temperatures because their materials are insulators and do not conduct the heat from the sun into the room.
4. The use of cloth to keep warm
Clothes keep us warm through holding air between the cloth and the body. It is not the clothes that warm the body but the air (a poor conductor) that the cloth traps around the body. Hence clothing made from woolen and fur materials are worn in the cold climates to retain heat and keep the body warm.
Convection
Heat can be transferred by convection in liquids and gases. Particles in the liquid or gas collide with a substance that has a high temperature and the particles gain kinetic energy. The particles move to a region which has substance at a lower temperature and the particles transfer heat by colliding with the colder substance. In this way the high temperature substance has heat taken away from it and gets colder. The low temperature substance has heat given to it and gets warmer. The liquid or gas that transfers the heat can circulate round and round between the hot and cold regions. The flow of liquid or gas is called convection current. Convection currents are caused by changes in density.
You may be asked to draw the direction of convection currents and explain why they move in this way.
The picture below shows a radiator heating a room in a house.
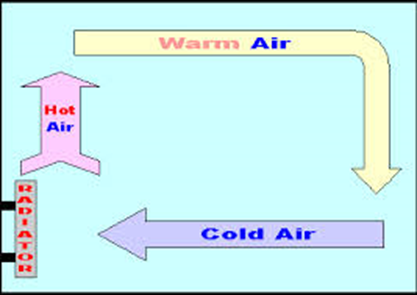
The hot radiator transfers heat to the nearby air when air molecules collide with the radiator surface.
The air is also heated by infra-red radiation. The hot air near to the radiator expands and increases in volume. Hot air expands because the particles move further apart as they get hotter (see the structure of a gas). This makes the density of the hot air decrease and it starts to rise upwards. The colder air above it gets pushed along to the right and then circulates as shown by the arrows. The arrows show how the convection currents move. As the hot air moves around the room, it loses its heat by collision with the walls, ceiling and the objects in the room. Finally the colder air circulates near to the radiator where it is heated and the whole process repeats itself. The efficiency of convection can be improved by placing shiny metal foil behind the radiator.
Convection current in Nature: Land and Sea Breezes
Land and sea breezes are the result of convection current in nature. In a hot day the sun warms the land more quickly than the sea, because the land is a better absorber of heat and also has a lower specific heat capacity. As a result, the air near the land warms up and rises. Cooler air from the sea moves in to replace the risen air. The convection current is completely by the air in the upper atmosphere moving down in the opposite direction.
The cool breeze that blows inland from the sea is known as a sea breeze.
At night, the reverse process takes place. The sea retains much of its heat in the night, while the land temperature drops considerably. As a result the sea is warmer than the land. Convection current is set up in the opposite direction to the daytime. The breeze from the land to the sea is known as land breeze.
Practical application of convection currents in cooling devices
1. Ventilation
Good ventilation in houses relies on the continuous circulation of convection air currents. Air heated by respiration and fires, rises towards the ceiling and escapes through the ventilators placed near the ceiling. This is replaced by fresh, cool air from outdoor which enters the room through the windows and other openings. In this way the room is ventilated.
2. Cooling of motor car engine
The motor car engine requires to be cooled to prevent overheating. Continuous convection current are utilized in the cooling process of the car engine. Water circulates round the engine by convection currents. The heat generated by the engine is conducted by the metal to the water in the jacket. The water itself is cooled by the draught of air created round the radiator by the motion of the car and the movement of the fan.
Radiation
Radiation is the process by which heat is transferred from a hotter to a cooler place without heating of the intervening medium.
Thus no material medium is required in radiation, but in conduction and convection, material medium is necessary. Heat from the sun reaches the earth by radiation. Also when you warm yourself by the fire side, the heat from the fire reaches by radiation.
Infra-red radiation (also called thermal radiation) transfers heat between all objects. The frequency at which an object emits electromagnetic radiation is called its principle frequency. The principle frequency increases as the temperature increases. The Sun is hotter than the Earth and so has a higher principle frequency. Infra-red radiation is an electromagnetic wave and can travel through a vacuum. Heat from the Sun reaches us through the vacuum of space by travelling as infra-red radiation.
An object can absorb radiation (take it in), emit radiation (give it out) and reflect radiation. The hotter an object, the faster it will emit infra-red radiation. Hotter objects will emit infra-red radiation faster than they absorb it from colder objects around them. Colder objects will absorb infra-red radiation faster than they emit it to hotter objects around them. In this way heat is transferred from hotter to colder objects.
An object whose temperature does not change emits infra-red radiation at the same rate as it is absorbed.
Objects that are at the same temperature as each other absorb, emit and reflect infra-red radiation at different rates depending on the type of surface that the object has.
Applications of radiation
Medical applications
The uses of radiation in diagnosis and treatment have multiplied so rapidly in recent years that one or another form of radiation is now indispensable in virtually every branch of medicine. The many forms of radiation that are used include electromagnetic waves of widely differing wavelengths (e.g., radio waves, visible light, ultraviolet radiation, X rays, and gamma rays), as well as particulate radiations of various types (e.g., electrons, fast neutrons, protons, alpha particles, and pi-mesons).
Imaging techniques
Advances in techniques for obtaining images of the body’s interior have greatly improved medical diagnosis. New imaging methods include various X-ray systems, positron emission tomography, and nuclear magnetic resonance imaging.
X-ray systems
In all such systems, a beam of X radiation is shot through the patient’s body, and the rays that pass through are recorded by a detection device. An image is produced by the differential absorption of the X-ray photons by the various structures of the body. For example, the bones absorb more photons than soft tissues; they thus cast the sharpest shadows, with the other body components (organs, muscles, etc.) producing shadows of varying intensity.
The conventional X-ray system produces an image of all structures in the path of the X-ray beam, so that a radiograph of, say, the lungs shows the ribs located in front and as well as in back. Such extraneous details often make it difficult for the physician examining the X-ray image to identify tumours or other abnormalities on the lungs. This problem has been largely eliminated by computerized tomographic (CT) scanning, which provides a cross-sectional image of the body part being scrutinized. Since its introduction in the 1970s, CT scanning, also called computerized axial tomography (CAT), has come to play a key role in the diagnosis and monitoring of many kinds of diseases and abnormalities.
In CT scanning a narrow beam of X rays is rotated around the patient, who is surrounded by several hundred X-ray photon detectors that measure the strength of the penetrating photons from many different angles. The X-ray data are analyzed, integrated, and reconstructed by a computer to produce images of plane sections through the body onto the screen of a television-like monitor. Computerized tomography enables more precise and rapid visualization and location of anatomic structures than has been possible with ordinary X-ray techniques. In many cases, lesions can be detected without resorting to exploratory surgery.
Positron emission tomography (PET)
This imaging technique permits physicians to determine patterns of blood flow, blood volume, oxygen perfusion, and various other physiological, metabolic, and immunologic parameters. It is used increasingly in diagnosis and research, especially of brain and heart functions.
PET involves the use of chemical compounds “labeled” with short-lived positron-emitting isotopes such as carbon-11 and nitrogen-13, positron cameras consisting of photomultiplier-scintillator detectors, and computerized tomographic reconstruction techniques. After an appropriately labeled compound has been injected into the body, quantitative measurements of its activity are made throughout the sections of the body being scanned by the detectors. As the radioisotope disintegrates, positrons are annihilated by electrons, giving rise to gamma rays that are detected simultaneously by the photomultiplier-scintillator combinations positioned on opposite sides of the patient.
Nuclear magnetic resonance (NMR) imaging
This method, also referred to as magnetic resonance imaging (MRI), involves the beaming of high-frequency radio waves into the patient’s body while it is subjected to a strong magnetic field. The nuclei of different atoms in the body absorb radio waves at different frequencies under the influence of the magnetic field. The NMR technique makes use of the fact that hydrogen nuclei (protons) respond to an applied radio frequency by reemitting radio waves of the same frequency. A computer analyzes the emissions from the hydrogen nuclei of water molecules in body tissues and constructs images of anatomic structures based on the concentrations of such nuclei. This use of proton density makes it possible to produce images of tissues that are comparable, and in some cases superior, in resolution and contrast to those obtained with CT scanning. Moreover, since macroscopic movement affects NMR signals, the method can be adapted to measure blood flow. The ability to image atoms of fluorine-19, phosphorus-31, and other elements besides hydrogen permit physicians and researchers to use the technique for various tracer studies as well.
Thermos flask – The Physics
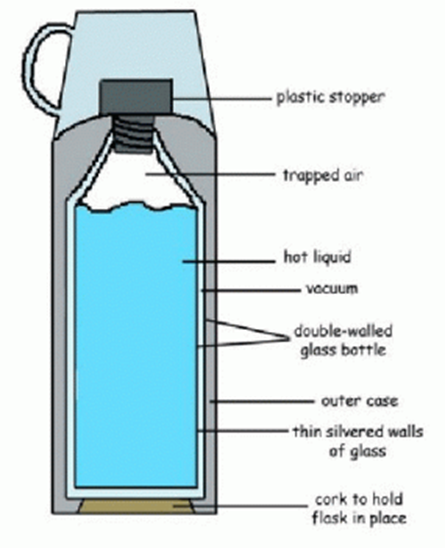
A thermos flask has double walls, which are evacuated and the vacuum bottle is silvered on the inside. The vacuum between the two walls prevents heat being transferred from the inside to the outside by conduction and convection. With very little air between the walls, there is almost no transfer of heat from the inner wall to the outer wall by convection. Conduction can only occur at the points where the two walls meet, at the top of the bottle and through an insulated support at the bottom.
The silvered walls reflect radiated heat back to the inside, the same way a space blanket does. The diagram below shows across section through a thermos.
Action
The students will examine the flask and explain how the three processes of heat transfer are affected by the flask. They should note that while the liquid inside is hot, and hence the inner wall is also hot, the outer wall is at approximately room temperature.
ASSESSMENT
- With respect to heat transfer through conduction, which of following inequalities place solids’, ‘liquids’ and ‘gases’ in right order?
(a) Solids < liquids < gases
(b) Solids < gases < liquids
(c) Solids > gases > liquids
(d) Solids > liquids > gases
- Which of following does not belong to list of factors that affect rate of transfer of energy by radiation?
(a) Color and texture of surface
(b) Temperature of surface
(c) Movement of air above surface
(d) Surface area - Rate of transfer of energy by radiation can be increased by
(a) increasing surface temperature
(b) decreasing surface area
(c) using shiny white surfaces instead of dull and black surfaces
(d) decreasing atmospheric pressure
- Process of heat transfer that involves continual emission of infrared waves from surface of bodies and transmission of these waves without aid of medium is known as
(a) Conduction
(b) Convection
(c) Radiation
(d) None of above - Vacuum in a vacuum flask prevents heat transfer through process of
(a) Conduction only
(b) Convection only
(c) Conduction and Convection
(d) Radiation only
ANSWERS
- d
- c
- a
- c
- c
ELECTRIC CHARGES PRODUCTION
WEEK 6
Topic: Production of Charges
1. Ensure your hair is dry, then rub the barrel of a plastic biro or ball pen vigorously on it. Hold the rubbed pen near some small pieces of paper and observe the effect of the pen on the paper.
2. Rub a plastic ruler vigorously against the sleeve of your shirt or blouse. Then hold the rubbed ruler over small pieces of paper and observe the effect on the paper.
3. Comb on a hair on a dry day with a plastic comb. Observe that tufts of hair will be attracted to various parts of the comb.
Repeat this activity in front of a mirror in a dark room and report your observations.
The rubbed plastic biro or ruler attracts small pieces of paper. The comb in the above experiment attracts some hair tufts and in dark room we can observe tiny sparks between the comb and hair especially during the dry harmattan season. Also during a dry day you can feel some crackling sensation as you comb your dry hair with a plastic comb.
The plastic passing through the dry hair as well as the hair itself acquire some electric charges by friction between the two and is said to be electrified or charged or to have a charge. Similarly the plastic biro or ruler rubbed vigorously on a shirt acquires electric charge by friction between the rulers and the shirt. These electrostatically charged materials attract the small pieces of, before attracting it. The piece of paper is also charged before being attracted.
There are other observations of static electricity in our daily experience. For example we often hear some crackling noise near the ear when taking off a dry sweater or a nylon garment especially during the dry harmattan weather. Also when the attempt to take off nylon or terylene clothes in a dry weather, the clothes tend to stick to the body because they are strongly electrified. The hair on our body has a strong pull on this types of clothes.
Types of Charges
There are two types of charges – positive and negative charges.
One type of electric charge could be produced by rubbing a glass rod with silk. This is the positive charge. A different type of charge could be obtained on an ebonite rod by rubbing it with fur. This type is known as the negative charge.
Rub a glass rod vigorously with silk and suspend it by a thread with a paper stirrup. Rob another glass rod by with silk and bring it near the first rod. Notice the repulsion between the two as the suspended glass rod moves away from the second glass rod.
In a similar fashion, rub an ebonite rod with fur and bring it near the suspended glass rod. Observe the attraction between the two rods.
Now, replace the suspended glass rod with an ebonite rod which has been charged by rubbing with fur. Repeat the previous experiment by bringing a charged ebonite rod and a charged glass rod in turn towards the suspended ebonite; observe that attraction and repulsion occur when the charged glass and ebonite rods are brought near the suspended charged ebonite rod.
ASSESSMENT
- Property of material due to which it attracts or repels other objects is
(a) friction
(b) velocity
(c) current
(d) charge - Plastic rod rubbed with fur and glass rod rubbed with silk
(a) repel each other
(b) mix up with each other
(c) attract each other
(d) none of the above
- A negative charge
(a) repels neutral charge
(b) attracts neutral charge
(c) repels negative charge
(d) repel positive charge - Electric charge between two bodies can be produced by
(a) sticking
(b) rubbing
(c) oiling
(d) passing AC current - If mica and woolen cloth are rubbed together, then mica gets
(a) positively charged
(b) negatively charged
(c) remains neutral
(d) dual charged
ANSWERS
- d
- c
- c
- b
- a
GOLD LEAF ELECTROSCOPE
Physics SS 1 WEEK 7
Topic: GOLD LEAF ELECTROSCOPE AND ITS USES, LIGHTNING CONDUCTOR
A gold leaf electroscope measures potential difference between the leaf and the base (or earth). The leaf rises because it is repelled by the stem (support). The leaf and its support have the same type of charge. A typical school electroscope will show a deflection for a charge as small as 0.01 pC (the unit pC is a pico coulomb, 1×10-12 coulombs, equivalent to the charge on over 6 million electrons).
Charging an electroscope
There are a number of ways of charging an electroscope. They include:
Charging by contact: Rub an insulator to charge it up. Then stroke it across the top plate of the electroscope. This will transfer charge from the insulator to the electroscope. This method is direct and clear to students. However, the charge left on the electroscope will not always leave it fully deflected.
Charging by induction: This is a quick way to get a larger charge onto the electroscope. However, it can look a bit magical to students. So it should be used with some care.
Rub an insulator to charge it up. Bring it close to the top plate of the electroscope – but don’t let it touch. This will induce the opposite charge on the plate of electroscope leaving a net charge on the gold leaf – which will rise. Now touch the plate with your finger momentarily to earth it (still holding the charged insulator near the top plate). The charge on the top plate will be neutralised but there will still be a charge on the gold leaf. Let go of the plate and then take the charged insulator away. The charge that had been pushed down to the gold leaf will now redistribute itself over the plate and the leaf, leaving the whole thing charged. The leaf will show a good deflection.
Detecting small currents
The electroscope can be used to demonstrate that a small current is flowing in a circuit – for example in experiments to show the ionisation of the air.
Using the hook rather than the plate makes the electroscope more sensitive to small amounts of charge. A charge of around 0.01 pC will cause a noticeable deflection of the gold leaf. So it is possible to watch it rise (or fall) slowly due to a current as small as 1 pA.
Put the electroscope in series (as though it were an ammeter). Any charge that flows in the circuit will move onto the electroscope making the gold leaf rise. You may need to discharge the electroscope when you first switch on the power supply because there will be an initial movement of charge due to the capacitance in the circuit.
Alternatively, you can use the electroscope as a source of charge and watch it discharge. It is like a capacitor with its own display. Charge it up and then connect it into a circuit. If the circuit conducts, the electroscope (capacitor) will discharge and, at the same time, the leaf will display how much charge is left.
Using the electroscope as a voltmeter or electrometer
The electroscope has a very high (as good as infinite) resistance. If you earth the electroscope case, the electroscope measures potential so it is well suited to detecting potentials in electrostatic experiments. Without earthing, the quantity it is measuring is charge. This is related to p.d. (by its capacitance C, i.e. V = Q/C). But it isn’t the same as p.d. because the capacitance can vary a lot – even during an experiment. Capacitance depends on the position of the electroscope, people nearby and so on.
So although the electroscope is useful as an indication of a voltage, it isn’t a reliable means of measuring it.
Cosmic radiation
School electroscopes are open to the air (more refined ones are in a vacuum). Cosmic radiation will ionise this air and cause a small leakage current. So the electroscope will discharge over time. Historically, the discharging of electroscopes led to the suggestion of the existence of cosmic radiation. Victor Hess and Carl Anderson shared the Nobel Prize for Physics in 1936, for discoveries related to cosmic radiation.
Lightning Conductors
A lightning conductor is a metal rod or metallic object mounted on top of a building, electrically bonded using a wire or electrical conductor to interface with ground or “earth” through an electrode, engineered to protect the building in the event of lightning strike. If lightning hits the building it will preferentially strike the rod and be conducted to ground through the wire, instead of passing through the building, where it could start a fire or cause electrocution.
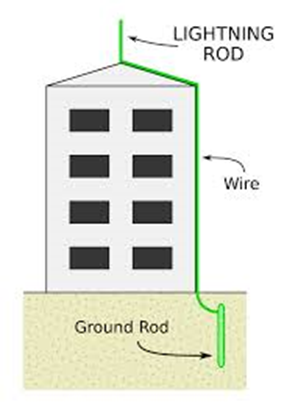
A lightning rod is a single component in a lightning protection system. Lightning rods are also called finials, air terminals or strike termination devices. The lighting rod requires a connection to earth to perform its protective function. Lightning rods come in many different forms, including hollow, solid, pointed, rounded, flat strips or even bristle brush-like. The main attribute of all lightning rods is they are conductive.
Copper and its alloys are the most common materials used in lightning protection.
Distribution of Charges on Conductors
Experimental works have shown that charges are distributed where there is a sharp curve. The density of these charges are greater at the surface of sharp curve. The sharp per unit area of a charged surface is called surface density. Surface density is greater at the corner or pointed edge than at the plain surface.
ASSESSMENT
- If body is positively or negatively charged leaves of electroscope will
(a) diverge
(b) converge
(c) stay still
(d) shrink - A sensitive instrument for detecting charges is called
(a) gold leaf electroscope
(b) telescope
(c) compound microscope
(d) chemical electroscope - Electroscope can be charged by
(a) convection
(b) radiation
(c) conduction
(d) both A and B - By using electroscope, if body is negatively charged then due to electrostatic induction, then on leaves there will be appear
(a) both positive and negative charges
(b) negative charge
(c) positive charge
(d) no charge - Divergence of leaves depends upon the
(a) current
(b) the amount of charge
(c) nature of leaves
(d) nature of disc
ANSWERS
- a
- a
- c
- b
- b
FIELD CONCEPT AND TYPES OF FIELD
Physics, SS 1 Week 8
Topic: FIELD CONCEPT AND TYPES OF FIELD
Concept of Fields
We define a field as a region of space under the influence of some physical agency such as gravitation, magnetism and electricity.
Force fields are forces whose sources do not require contact with the body to which they are applied. We identify such force fields as gravitational force, electric force, magnetic force and electromagnetic force.
There are two classes of force fields – scalar fields and vector fields. A scalar fields is the one that has only magnitude but no direction, e.g. temperature, energy and density. A vector field is a field that has both magnitude and direction, e.g. gravitational, magnetic and electric fields.
Gravitational Field
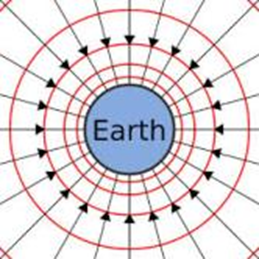
If we throw up massive objects, it is our common observation that they move up to their highest points, stay stationary very briefly and eventually move downwards, falling faster and faster until they hit the ground level or the lowest level on their path. The up and down movements of objects on the earth’s surface are subject to the influence of the Gravitational field of the earth.
Gravitational field is a convenient and informative method of the describing the gravitational attraction of one body for another at a distance. Gravitational field is a force field and influences the motion of objects in the space where it operates, without coming in contact with the objects. Gravitational field acts over a distance, it surrounds every object that has mass and its permeates all of space.
The earth attracts every object existing in the earth’s gravitational field. This attraction is called gravitational attraction and its effect is to change the velocity of objects under its influence, i.e. to accelerate such objects.
The acceleration of objects due to the earth’s gravitational attraction is called the acceleration due to gravity. It is represented by the symbol g whose average value is about 9.81 ms-2.
The acceleration due to gravity g is uniform at a given place and is the same for all bodies irrespective of their masses. It however varies from place to place. It is minimum at the equator (9.78 ms-2) and increase with latitude reaching the maximum value o f9.83 ms-2 at the poles of the earth. Hence it is less in Lagos than in London.
It is because the acceleration due to gravity is the same for all bodies in the same locality that all objects whatever their masses, when released from rest at the same point above the ground fall to the ground simultaneously (at the same time).
The force of gravitational attraction is given by F = mg, where g is the acceleration due to gravity and m is the mass of the object. When m = 1, F = g. This is known as the Acceleration of free fall (g) due to gravity. This is the force of attraction on a unit mass.
All bodies at the same place in its earth’s gravitational field are accelerated at the same rate, g, due to earth’s gravitational attraction. We should therefore expect a stone and a feather or a sheet of paper, when dropped from the same height to reach the ground at the same time. This is not however the observation, as the stone is seen to fall faster and thus takes a shorter time to reach the ground than the feather.
This is due to air friction or resistance which retards the movement of the feather more than that of the heavier stone. Due to its large surface area, the feather falls more slowly than the stone because of the large amount of air resistance (i.e. in a vacuum) both the heavier stone and the lighter feather will reach the ground at the same time.
In the absence of air resistance or friction all bodies fall with the same acceleration irrespective of their masses.
The strength of the gravitational field is smaller on the moon than on the Earth. So an astronaut has a lower weight on the Moon than on the Earth. The force pulling him down is smaller, so he can move easily on the Moon.
Magnets
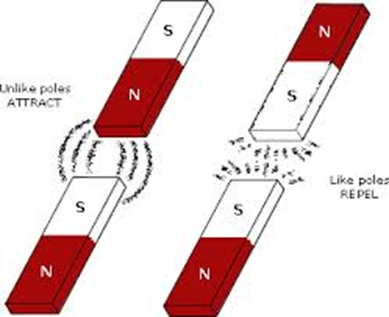
The pole of a magnet is the proportion of the magnet where its magnetic attraction appears to be strongest.
A magnet can attract pieces of iron, nickel and cobalt. Such substances that can be attracted by a magnet are known as magnetic substances ad this ability of a magnet attract magnet substances is called magnetism.
A bar magnet suspended about its centre in such a way as to swing freely, quickly comes to rest with its axis aligned approximately in the North-South direction.
The end of the magnet that points in the northward direction is the North pole. The other end that points approximately in the southward direction is the South Pole. If we bring the north pole of another magnet near the north pole of the suspended magnet, we will notice that the suspended magnet moves away from the new magnet. Thus there appear to be a force of repulsion between the two north poles of these magnets. Similarly a south pole of a magnet brought near the south pole of the suspended magnet will repel it.
Now we can reverse this experiment and bring the north pole of a magnet near the south pole of the suspended magnet. We will notice that the two poles now attract each other. The same attraction is noticed when south pole is brought near the north pole of the suspended magnet. We therefore conclude that:
Like or similar poles of magnets repel one another but unlike or dissimilar poles attract one another.
Magnetic Field
The region around a magnet in which the influence of the magnet can be felt or detected is called a magnetic field.
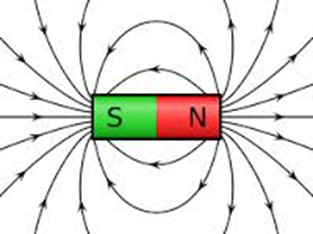
This space can be mapped out using a magnetic compass needle. The compass needle when placed in a magnetic field swings around and settled in a definite direction. The swinging of the needle when in the vicinity of the magnet shows that it has been subjected to a force. The force is known as the magnetic field. Thus, magnetic field is a force field. Magnetic force can be felt at a distance. It influences an object even when not in contact with it.
Magnetic field is also a vector field, i.e. it has both magnitude and direction.
We can demonstrate the pattern or nature of the magnetic field around a bar magnet by use of (a) iron fillings (b) magnetic compass needle.
ASSESSMENT
- Electric field intensity is
(a) a base quantity
(b) a scaler quantity
(c) A and B both
(d) a vector quantity - Field lines always emerge from
(a) negative charge
(b) positive charge
(c) can be both charges
(d) the central point of both charges - Direction of free test charge will be
(a) direction of electric intensity
(b) direction of coulomb’s force
(c) direction of magnetic intensity
(d) direction of protons - Spacing between field lines shows
(a) their direction
(b) their position
(c) both A and B
(d) their strength - Region around a charge q in which it exerts force on a test charge is called
(a) electric field intensity
(b) electric force
(c) electric field
(d) coulomb’s force
ANSWERS
- d
- b
- a
- d
- c
ELECTRIC FIELD
Physics, SS 1 Week 9
Topic: ELECTRIC FIELD
An electric field is a region of space where a charged body experiences an electric force.
The electric field is just a way of describing the action at a distance of one charge upon another. Such a field is another example of a force field and is represented by electric lines of force. Since force is a vector quantity, an electric force field has both magnitude and direction.
The direction of an electric field at any point is given by direction of the force acting on a small positive charge placed at that point.
There are two lines of fields –
a. uniform field, in which the field lines are straight,
b. variable field, in which the field lines are curved.
Electric line of force is an imaginary line drawn in an electric field in such a way that the direction at any point (or the direction of the tangent) gives the direction of the electric field at such a point. OR, the path which an isolated small positive charge would follow if placed in the field.
Patterns of electric field
The field about an isolated positive charge is radially outward, because a positive charge placed at any point around it is repelled outward along a line through the two charges.
Similarly the field about a negative charge is radially inwards as shown. The fields for other charges or a combination of charges as shown below.
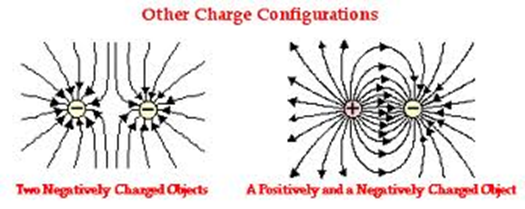
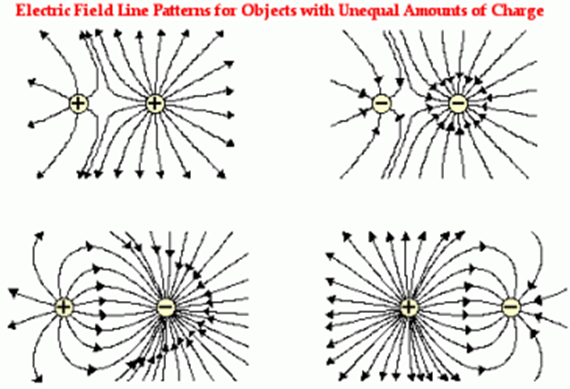
Properties of Electric Lines of Force or Field Lines
1. The electric lines of force are imaginary lines.
2. A unit positive charge placed in the electric field tends to follow a path along the field line if it is free to do so.
3. The electric lines of force emanate from a positive charge and terminate on a negative charge.
4. The tangent to an electric field line at any point gives the direction of the electric field at that point.
5. Two electric lines of force can never cross each other. If they do, then at the point of intersection, there will be two tangents. It means there are two values of the electric field at that point, which is not possible.
Further, electric field being a vector quantity, there can be only one resultant field at the given point, represented by one tangent at the given point for the given line of force.
6. Electric lines of force are closer (crowded) where the electric field is stronger and the lines spread out where the electric field is weaker.
7. Electric lines of force are perpendicular to the surface of a positively or negatively charged body.
8. Electric lines of force contract lengthwise to represent attraction between two unlike charges.
9. Electric lines of force exert lateral (sideways) pressure to represent repulsion between two like charges.
10.The number of lines per unit cross – sectional area perpendicular to the field lines (i.e. density of lines of force) is directly proportional to the magnitude of the intensity of electric field in that region.
11. Electric lines of force do not pass through a conductor. Hence, the interior of the conductor is free from the influence of the electric field.
12. Electric lines of force can pass through an insulator.
ASSESSMENT
- Particles involved in movement within material are
(a) protons
(b) electrons
(c) neutrons
(d) positrons - Phenomena in which a charged body attract uncharged body is called
(a) electrostatic induction
(b) electric current
(c) charge movement
(d) magnetic induction - An uncharged object has
(a) more protons
(b) more electrons
(c) equal electrons and protons
(d) no protons and electrons - Fields that act on objects with masses are
(a) electric fields
(b) magnetic fields
(c) force fields
(d) gravitational fields - Where an electric charge experiences a force, there is an
(a) electric field
(b) magnetic field
(c) electric current
(d) conventional current
ANSWERS
- b
- a
- c
- d
- a
PRODUCTION OF CONTINUOUS ELECTRIC CURRENT, CHEMICAL ENERGY, HEAT ENERGY, MECHANICAL ENERGY, SOLAR ENERGY
Physics SS 1 Week 10
Topic: PRODUCTION OF CONTINUOUS ELECTRIC CURRENT, CHEMICAL ENERGY, HEAT ENERGY, MECHANICAL ENERGY, SOLAR ENERGY
Production of Electric Current
Electric current or a continuous flow of charge can be generated from (a) chemical energy (b) heat energy (c) mechanical energy (d) solar energy.
Electricity is produced from chemical energy through the use of electric cells. A cell is a device for converting chemical energy into electrical energy.
Electricity from chemical energy
Electricity is produced from chemical energy through the use of electric cells. A cell is a device for converting chemical energy into electrical energy.
A cell consists of two dissimilar (or unlike) metals separated by solutions of various acids or salts. The metals are known as the anode, the negative electrode is the cathode. A simple cell consists of a copper rod and a zinc plate immersed in a container filled with dilute sulphuric acid (tetraoxosulphate (VI) acid).
A simple cell consists of a copper rod and a zinc plate immersed in a container filled with dilute sulphuric acid (tetraoxosulphate (VI) acid).
When the copper rod and zinc plate are joined by a conductor (e.g. a metallic wire), the zinc slowly dissolves in the acid and bubbles of hydrogen gas are formed on the copper rod. At the same time as a result of chemical reaction, electrons flow through the connecting wire from zinc to copper. Copper is the anode (+ve electrode). Zinc is the cathode (-ve electrode). By convention, current is taken to flow from copper to zinc even through the electrons move in opposite direction from zinc to copper. A bulb connected between the copper and zinc terminals will light up, showing that current is showing through the external circuit.
Electricity from heat energy (The thermoelectric effect)
We can show that heat energy can be converted into electric currents by joining two different metallic wires (e.g. copper and iron) at one end and connecting the free ends to the terminals of a sensitive current detecting device, e.g. a milli-galvanometer. When the junction of the metals is put in hot water, a current is observed to flow along the wires as indicator by the milli-galvanometer. The junction placed in hot wire is known as the hot junction, while the ends connected to the instrument constitute the cold junction.
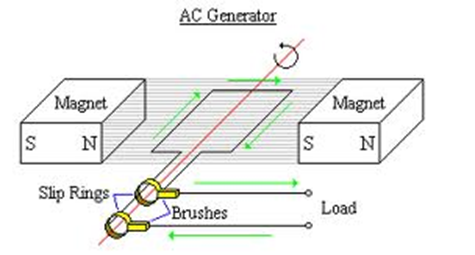
The greater the difference in temperature between the hot and cold junctions, the greater the current flow.
The two metal joined in this way constitute a device known as a thermocouple and the electricity (known as thermoelectricity)is produced by a process known as the thermoelectric effect. The current produced by the thermoelectric effect is small and can only be detected by very sensitive galvanometers.
Electricity from mechanical energy
A great majority of the world’s electricity is produced from the conversion of mechanical energy into electrical energy. When coils of insulated wire move across the magnetic field between two magnets, current is induced in the coils because the coils cut across the magnetic lines of force. The current is tapped using split-ring commutators and carbon brushes.
The electric generator or dynamo produces electricity in this way. A common example of such device is the bicycle dynamo used to supply light to the headlamp of a bicycle.
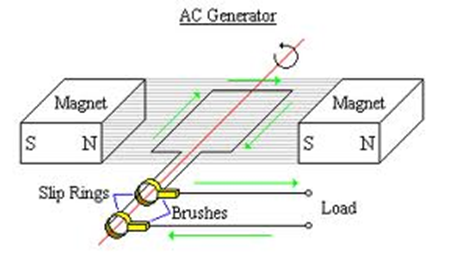
Electricity from solar energy
When sunlight falls on a photosensitive surface (e.g. the surface of potassium, an alkaline metal), electron are produced whose movements constitute a current. A photoelectric cell or photocell consists of a photosensitive surface as a cathode and a wire ring as the anode. If visible light falls on this surface, electrons are emitted by the photoelectric effect and the floe of these electrons can be detected as current by a micrometer.
The electrons are usually accelerated from the cathode to the anode which is at a higher potential with respect to the cathode. Solar electricity can be used to light up a whole village.
ASSESSMENT
- A boulder sitting on top of a mountain has ________ energy.
(a) mechanical
(b) kinetic
(c) mechanical potential
(d) chemical electrical - The form of energy found in food, wood, and batteries is _______ energy.
(a) chemical
(b) mechanical kinetic
(c) electrical
(d) solar - When you move your arm from side to side, your body changes
(a) chemical energy into mechanical kinetic energy
(b) heat energy into electrical
(c) mechanical potential energy into heat energy - Burning wood in a fireplace transforms ________ energy into ________ energy.
(a) sound, light
(b) chemical, heat and light
(c) light, heat
(d) mechanical kinetic, mechanical potential - The sun causes a tomato plant to produce tomatoes through a process called photosynthesis. What energy transformation best explains this process?
(a) solar to heat
(b) solar to chemical
(c) solar to electrical
(d) solar to mechanical kinetic
ANSWERS
- c
- a
- a
- b
- b